Recent Advances in Ergothioneine
Explore the latest insights on ergothioneine’s role in health, disease prevention, and therapeutic applications, highlighting key transport mechanisms and biosynthesis.
Abstract
Recently, there has been a surge of interest in a unique low-molecular-weight dietary thiol/thione, ergothioneine. This compound can accumulate at high levels in the body from dietary sources and may play a significant physiological role in human health and development, as well as in the prevention and treatment of diseases. Levels of ergothioneine in the blood decrease with age and the onset of various diseases. Here, we highlight the latest advancements in our understanding of ergothioneine in the human body.
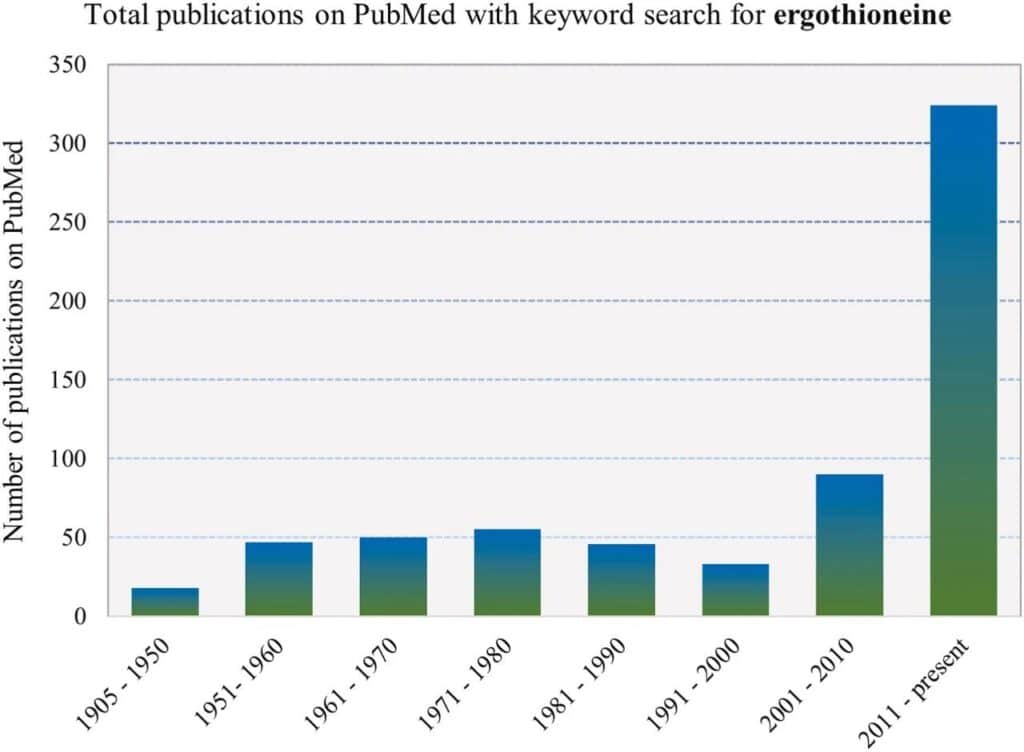
Introduction
The unique sulfur chemistry of thiols plays a vital role in cellular function and the protection of cellular components. The body has evolved to rely on a rich array of low-molecular-weight thiols, such as glutathione (GSH), to maintain redox balance and also serve other critical roles including xenobiotic detoxification and signaling. Some of these thiols can also be derived from the diet, like ergothioneine (ET), a trimethylbetaine derivative of histidine. Rediscovered a century after its initial discovery, interest in this low-molecular-weight thiol/thione has been reignited partly due to its unique chemical properties (e.g., unusual stability) and the identification of a transporter, Organic Cation Transporter Novel Type 1 (OCTN1), responsible for the uptake and accumulation of ET in human tissues from our diet and its selective distribution due to varied expression of the transporter. Recent attention to ET is reflected by the exponential growth in publications mentioning this compound (Figure 1). These include extensive studies revealing the relationship between ET levels in the blood and health and disease, cellular and tissue protective mechanisms, potential therapeutic applications of ET, expression and tissue distribution of the transporter, biosynthetic mechanisms, etc. This review emphasizes new insights and recent discoveries regarding this remarkable compound.
Ergothioneine Transporters
Organic Cation Transporter Novel Type 1, the ergothioneine transporter, was discovered in 2005 (encoded by the gene slc22a4) to be primarily a transporter of ET, exhibiting higher transport efficiency than many other related metabolites. Without it (e.g., in knockout animal models), there is no ET in cells and tissues. From its initial concept as primarily an ET transporter, many other studies have shown that OCTN1 also participates in the transport of nucleosides, acetylcholine, tetraethylammonium, spermine, L-carnitine, cytarabine, gemcitabine, gabapentin, oxaliplatin, and metformin. However, many of these claims utilized non-conventional models far from physiology. Recently, using naturally expressed and over-expressed OCTN1 in human cells, the selective preference of ET as a substrate for OCTN1 was reaffirmed, supporting the suggestion to rename OCTN1 as the Ergothioneine Transporter (ET). However, since most literature still uses the term OCTN1, we will adhere to it in this article.
This transporter is responsible for the substantial dietary uptake of ET, widely distributed across many tissues (Figure 2). ET is found in the tissues of most laboratory rats and mice, although their diets contain only trace amounts of ET, suggesting effective uptake and retention of this nutrient. OCTN1’s expression is also significant in the proximal renal tubules, important for renal absorption of ET, which may promote its accumulation in the body. Indeed, compared to wild-type animals (<10%), OCTN1 knockout mice administered ET intravenously showed a higher urinary excretion rate (>50% of the administered dose). The ability of ET to accumulate in the body and its selective distribution in tissues (through differential expression of transporters) suggest that ET plays a significant physiological role. However, OCTN1 knockout models (lacking tissue ET) have yet to show any substantial dominant phenotypes or defect syndromes. This might be due to compensatory defense pathways maintaining homeostasis in healthy animals, although the phenotype becomes more apparent under stress. The consequences of ET deficiency are hard to demonstrate in humans due to its abundant presence in the diet and strong retention by the human body. However, lower blood ET levels are associated with the incidence of diseases such as Parkinson’s disease (PD), Mild Cognitive Impairment (MCI), Crohn’s disease (CD), and frailty, while correspondingly higher blood ET levels are associated with lower incidence of cardiac metabolic disorders and related mortality and peripheral neuropathy. This aligns with the view that ET deficiency might increase disease risk. Whether a decline in ET is a cause or consequence of disease remains to be determined by further longitudinal studies.
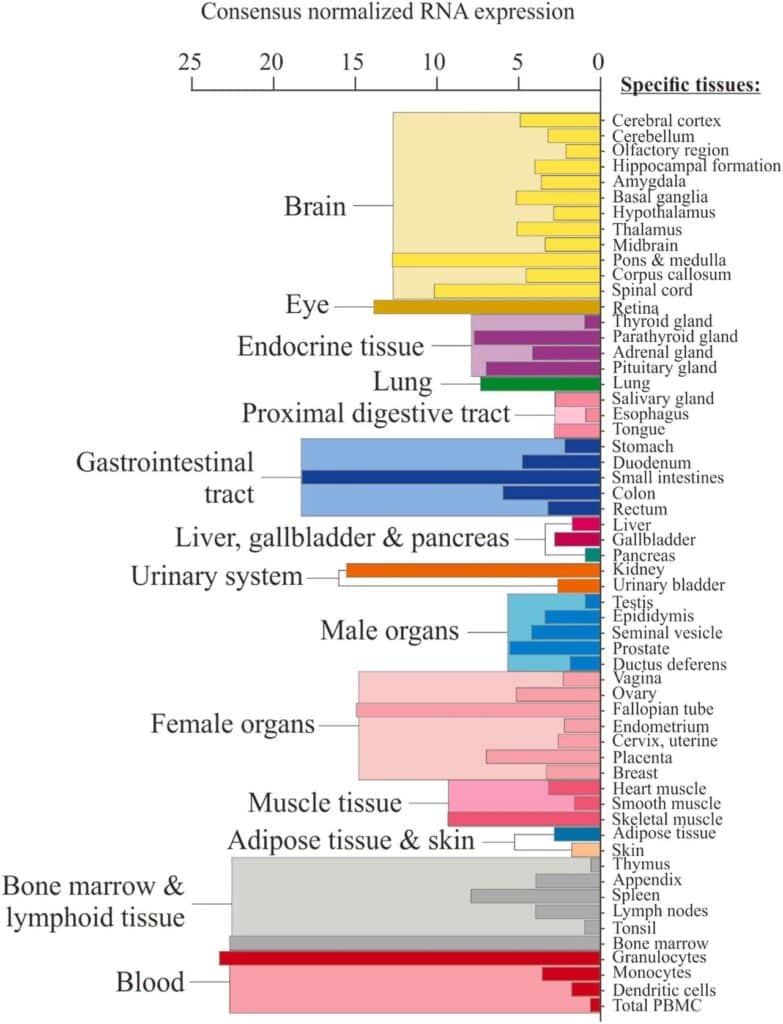
Factors Influencing Human Ergothioneine Transport
ET can be found in most tissues (if not all), with varying accumulation rates due to differential transporter expression. In some cells, high expression of the transporter can be observed, therefore high levels of ET (e.g., in blood cells, bone marrow, oc
ular tissues, the brain, etc., Figure 2) may be susceptible to oxidative stress, although other tissues can accumulate high levels of ET through sustained administration. In certain animal disease models, such as fatty liver disease, CD, and chronic kidney disease, OCTN1 transporters (via transcriptional activators including RUNX1, Sp1, and NFκB) are elevated due to tissue damage. This is considered an adaptive physiological response that can increase ET levels in damaged tissues, thereby limiting further damage. While ET is obtained from the diet, studies suggest that tissue levels of ET closely correlate with OCTN1 expression or transporter polymorphisms; populations exhibit many OCTN1 single nucleotide polymorphisms (SNPs), which affect ET transport kinetics. Supporting this view, individuals with higher baseline blood ET levels also exhibit greater ET uptake and accumulation after oral administration, possibly due to transporter polymorphism. However, it is currently unclear if OCTN1 polymorphisms (possibly explaining lower blood ET levels) may predispose certain individuals to disease. The authors suggest that these variants, leading to up to a 50% efficiency and threefold affinity increase for ET, are positive mutations caused by lower ET in the diet of these historical populations or high UV exposure of ancient farmers in the region (see Section 3.2.6). Claims of a link between OCTN1 polymorphisms and IBD or rheumatoid arthritis remain controversial. Longitudinal studies of OCTN1 SNPs in populations, their blood ET levels, and their relationship with disease should provide further insights.
Cellular Localization
Early studies suggested (but did not rigorously determine) that OCTN1 is present in mitochondria, allowing ET to accumulate in this organelle. Indeed, ET can protect mitochondrial DNA from oxidative damage caused by hydrogen peroxide or UV irradiation. Since then, few studies have investigated the intracellular localization of OCTN1 and ET. Mitochondrial dysfunction is associated with multiple diseases, and the therapeutic benefits of ET are thought to be mediated by protecting this organelle. We recently confirmed that ET could protect mitochondrial morphology in a transgenic fruit fly model of PD. More definitive evidence of ET uptake and accumulation in mitochondria is needed.
Another Transporter?
A recent study on an isolated solute carrier (part of the superfamily to which OCTN1 belongs), SLC22A15, suggests that it can transport ET into transfected HEK293 cells, although its transport efficiency is significantly lower than OCTN1. The authors believe that SLC22A15’s expression in the brain is higher than OCTN1, making it potentially important for ET deposition in the brain or possibly translocation in the BBB, although the expression levels of OCTN1 remain uncertain. However, SLC22A15 does not seem to be involved in the intake of ET from the diet into the body, as OCTN1 knockout mice show no notable ET levels in the blood and tissues after oral administration. Indeed, SLC22A15 is expressed at lower levels in the gastrointestinal tract. However, when ET is injected intravenously, the brain levels in OCTN1 knockout and wild-type mice seem comparable, albeit lower. Further research is needed to determine whether SLC22A15 indeed participates in the brain uptake and accumulation of ET.
Sources of Ergothioneine
From a Culinary Perspective
ET is present in various foods, but the highest dietary levels of ET are found in certain mushrooms and spirulina, which produce their own ET. Some studies have investigated the potential health benefits of consuming mushrooms. These factors include increased mushroom intake associated with dementia, cardiovascular diseases, some cancers (such as prostate and breast cancer), metabolic syndrome, PD, and other neurological diseases, viral infections, and many other diseases. Some studies attribute these health benefits to the present ET. However, mushrooms also contain many other compounds (such as β-glucans, polysaccharides, etc.) that may also be beneficial.
Gut Microbiome and ET Biosynthesis?
The gut microbiome plays a key role in human health and disease. This vast microbial repository, encoding 100 times more genes than the host, is responsible for some essential metabolic functions, including the production of certain vitamins and amino acids. While animals and humans can only obtain ET from dietary sources, certain bacteria can synthesize ET, including members of the Actinobacteria and Betaproteobacteria phyla. They produce ET from histidine, although through different mechanisms. Transforming E. coli (naturally incapable of ET biosynthesis) with egtBD genes enabled them to produce ET, although adding egtACE genes increased E. coli’s ET production. A thorough analysis of the normal gut microbiome’s bacteria, actinobacteria, cyanobacteria, and proteobacteria revealed that many of these bacteria appear to have egtBD genes, or their homologs. This raises the question of whether the gut microbiome can produce ET. Despite evidence suggesting this might not be
the case, this may need to be re-examined using more sensitive and accurate ET analysis methods. If so, ET produced by the microbiome could contribute to tissue levels of ET, and changes in the gut microbiome might affect body ET levels as seen in many diseases. This could also offer another explanation for lower blood ET levels seen in many diseases. Indeed, as more evidence emerges of a complex connection between the gut microbiome and the brain, changes in the microbiome leading to reduced body ET levels could lead to disease. So far, no studies have determined that the gut microbiome can produce ET, but one study suggested that Lactobacillus reuteri might produce ET in vitro, and L. reuteri disease was associated with increased fecal ET levels in rats. However, the presence of ET in microbes is not definitive evidence of ET production, as some bacteria can absorb ET from their environment. Research using isotopically labeled precursors or isolation of ET biosynthetic enzymes is needed to confirm ET production. The authors indeed found that elevated fecal ET, in this model using ET, improved sleep disturbances and social avoidance behaviors. However, our laboratory’s studies using isotopically labeled histidine as a precursor showed no signs of ET biosynthesis. L. reuteri, and the genomes of lactic acid bacteria seem not to contain ET biosynthetic genes. Perhaps the authors considered that lactic acid bacteria could accumulate ET from their environment. However, the gut microbiome’s uptake and utilization of ET may still play a key role in the host’s intake of ET. More work is needed to study this.
Indeed, E. coli can break down ET to produce thiourea and trimethylamine, attributed to trimethylamine-cleaving enzymes and ergothioneinases. Other microbes, including Burkholderia and Spirochaetes and Faecalibacterium, also possess ergothioneinases. Further research is needed to assess whether other commensal bacteria can degrade ET, and whether this helps reduce gut absorption of ET.
Biosynthetic Mechanisms
The European Food Safety Authority has already allowed the use of ET as a food additive and supplement, even for pregnant women and infants. At the same time, the US Food and Drug Administration has granted ET “Generally Recognized as Safe” (GRAS) status as a food additive and supplement. This facilitates ET’s broader application in general health and well-being, or in combating a range of diseases. In addition to chemical synthesis, several groups have demonstrated the biosynthesis of ET by yeast, with ET bioproduction in brewing yeast through cloning mushroom’s two ET biosynthetic genes or combinations of bacterial and fungal genes from Mycobacterium smegmatis and fungi. Other research groups have enhanced ET biosynthetic gene copy numbers and increased the availability of the histidine precursor through recombinant methods. Other approaches have also been used to utilize synthetic biology to produce ET, employing a range of bacterial and fungal microbes to expand its production, even for the production of isotopically labeled ET variants for research purposes.
Ergothioneine in Infectious Diseases
2019 Coronavirus Disease (COVID-19)
The highly contagious severe acute respiratory syndrome coronavirus that caused the COVID-19 pandemic has infected over 100 million people and resulted in nearly 2.2 million deaths worldwide (WHO COVID-19 Situation Report) as of the writing of this article. The struggle to contain coronavirus infection has produced changed uses and new treatment methods to reduce the severity and duration of the disease. We believe ET might alleviate symptoms and improve prognosis in COVID-19 patients because it can prevent potential COVID-19 pathologies, such as acute respiratory distress syndrome (the main cause of death in COVID-19), excessive oxidative damage, inflammation, and endothelial dysfunction. ET’s presence in major organs can also protect them from damage due to excessive immune responses and prevent permanent damage (such as fibrosis).
Opportunistic Pathogens
The dark side of ET is that some pathogens can synthesize ET, including Burkholderia and Mycobacterium tuberculosis, as a means of protecting host defenses or enhancing antibiotic resistance. Indeed, in Mycobacterium tuberculosis that causes tuberculosis, ET maintains bioenergetic homeostasis and protects against oxidative stress, enhancing toxicity in animal models. Opportunistic fungal pathogens A also have ET biosynthetic capability, which might enhance their resistance to environmental heavy metals and host-produced ROS.
As previously mentioned, although most microbes cannot perform ET biosynthesis, some can accumulate and utilize ET from their environment, especially some opportunistic pathogens [4]. Like Escherichia coli] and oral pathogen Treponema denticola. Therefore, drugs targeting uptake mechanisms might help limit their pathogenicity. A clearer understanding of ET’s role in pathogenicity could reveal mechanisms to reduce toxicity and/or resistance.
Alternative Applications of Ergothioneine
Given ET’s unique chemical properties, it’s no surprise that it has numerous alternative applications as well. S
-methyl ergothioneine and haixi alkaloid have been identified as potential metabolites of ET in the body. Indeed, their levels are highly correlated with ET levels in the blood and most tissues (although there are some exceptions like usually higher levels of haixi alkaloid in the brain). However, their functions are not yet clear. Glutathione S-transferases (GST) utilize GSH to catalyze the detoxification of various xenobiotics and might facilitate GSH methylation. Whether another enzyme like this exists or if GST can also utilize ET is currently unclear. A study described ET as a xenobiotic amino acid, promoting nucleophilic substitution-type reactions to detoxify toxins in vitro in a manner similar to GSH, demonstrated with the formation of an ET-CDNB product in human lung epithelial cells, enhancing cell viability. Although the involved enzymes are not yet clear, the ET-CDNB product might be exported from cells via multidrug resistance transporters. Further research is needed to validate this and clarify the involved enzymes. ET is also used as a food preservative. Edible mushroom extracts can prevent melanosis or black spot formation in harvested shrimp, attributed to ET’s anti-melanin and antioxidant properties. Similarly, studies have shown that ET can prevent lipid and myoglobin oxidation in beef and fish, hence, patents have been applied for ET as a food, drug, and beverage preservative in the US and Canada (ET20130035361A1 and CA2680223A1 and 201A1 and CA2680223A1). Moreover, ET’s unique chemical properties might enable various fluorophores to have strong photostability, and recent research has also shown that ET has intense surface-enhanced Raman scattering spectra, which the authors believe could make non-invasive analysis of ET in biological fluids a predictive tool. Interestingly, ET has also been suggested as a metabolite to estimate the age of bloodstains at crime scenes, due to its stability and slow time-dependent degradation.
In Conclusion
ET’s unique chemical properties, with its high stability and the ability to accumulate in the body, endow it with extensive cellular protective and disease mitigating properties. Although low blood levels of ET are linked to various human diseases, there is still much unknown information. However, the intake in fetuses and infants (if not complete), the ubiquitous presence in tissues, and the body’s strong retention are compelling evidence of ET’s critical role in human development and health. Many animal studies have also demonstrated its benefits, and blocking uptake through silencing OCTN1 seems to worsen the pathology in these disease models. Whether humans, especially older individuals or those with lower blood ET levels (due to transporter or dietary polymorphic variants), can reduce the risk of age-related diseases and other ailments, or take actions to slow or prevent disease progression as a therapeutic agent remains to be observed. The safety profile of ET and regulatory body approvals help facilitate this type of research. With rapidly increasing interest (Figure 1), it’s undoubtedly an exciting time for this unique low-molecular-weight compound.
About ETprotein:
ETprotein, a reputable protein and L-(+)-Ergothioneine (EGT) Chinese factory manufacturer and supplier, is renowned for producing, stocking, exporting, and delivering the highest quality organic bulk vegan proteins and L-(+)-Ergothioneine. They include Organic rice protein, clear rice protein, pea protein, clear pea protein, watermelon seed protein, pumpkin seed protein, sunflower seed protein, mung bean protein, peanut protein, and L-(+)-Ergothioneine EGT Pharmaceutical grade, L-(+)-Ergothioneine EGT food grade, L-(+)-Ergothioneine EGT cosmetic grade, L-(+)-Ergothioneine EGT reference grade and L-(+)-Ergothioneine EGT standard. Their offerings, characterized by a neutral taste, non-GMO, allergen-free attributes, with L-(+)-Ergothioneine purity over 98%, 99%, cater to a diverse range of industries. They serve nutraceutical, pharmaceutical, cosmeceutical, veterinary, as well as food and beverage finished product distributors, traders, and manufacturers across Europe, USA, Canada, Australia, Thailand, Japan, Korea, Brazil, and Chile, among others.
ETprotein specialization includes exporting and delivering tailor-made protein powder and finished nutritional supplements. Their extensive product range covers sectors like Food and Beverage, Sports Nutrition, Weight Management, Dietary Supplements, Health and Wellness Products, and Infant Formula, ensuring comprehensive solutions to meet all your protein needs.
As a trusted company by leading global food and beverage brands and Fortune 500 companies, ETprotein reinforces China’s reputation in the global arena. For more information or to sample their products, please contact them and email sales(at)ETprotein.com today.